The following is reprinted from The International
Trumpet Guild Journal, Vol. 20, No. 3 Feb, 1996.
Japanese
Francais
The Asymmetric
Trumpet Mouthpiece JOHN LYNCH
Over the
years numerous attempts have been made to improve the
performance of trumpet mouthpieces in specific areas such as
ease of playing, more desirable tone, and easier high
register. These efforts have been, to my knowledge,
essentially trial and error approaches, wherein improvement is
determined by soliciting the opinions of various musicians.
This approach to mouthpiece development has had limited
success. Some progress has been made from these empirical
activities, but only a few generalizations have emerged that
appear to hold true. Two of these, which are usually regarded
as "rules-of-thumb" and are widely accepted among mouthpiece
manufacturers and trumpet players alike, are:
- A
mouthpiece having a shallow (low volume) cup allows higher
notes to be played more easily but produces a more metallic
tone quality throughout the complete range of the
instrument. Shallow cup mouthpieces are, therefore,
desirable for the former property and undesirable for the
latter.
- A
mouthpiece having a deep (high volume) cup produces a more
desirable tone but is frequently difficult to play in the
extreme high register. Deep cup mouthpieces are, therefore,
desirable for the former property and undesirable for the
latter.
These
rules have led to two distinct approaches to playing the
trumpet. The more common approach, adopted by many players, is
to use a mouthpiece having a cup of intermediate depth as a
compromise. The other approach has been to use either a very
deep cup mouthpiece or a very shallow cup mouthpiece,
depending on the type of performance required by the
particular performer; i.e., if all his performances require
extreme high-register playing, he will use a very shallow cup
mouthpiece and accept the brassy sound in the lower register.
But if all his performances don't require extreme
high-register playing, he will use a deep cup mouthpiece in
order to obtain a more sonorous and desirable sound. These
have been and are, the traditional approaches to mouthpiece
selection, and both leave much to be desired.
In the
case of the player who chooses the compromise of medium-depth
cup, such a compromise usually produces a player of limited
ability as an altissimo player and one whose tone quality is
also somewhat less than ideal. And, for the player who bases
his selection of mouthpiece cup depth either toward a very
shallow or a very deep cup, similar limiations are seen in
either high register-capability or tone quality. These
limiations are a problem, because a performer's lips must
become acclimated to a change in mouthpieces; this acclimation
can require only a few days, but in some cases it may take
weeks. Therefore, it is not generally feasible to change
mouthpieces from one cup design to another to suit the
immediate demands of the music. Thus, presently available
mouthpieces do not offer trumpet players an effective solution
to either range or tone problems.
In
addition to these difficulties, others are fundamental to
trumpet playing in particular. One is the great physical
effort that must be exerted at and around any player's
particular high limit. Another is that even the shallowest
available mouthpiece can only be reliably played by many
advanced student players, some of whom are proficient players
in other respects, up to a modestly high limit of about C'''.
Tumpet players today however, are expected to be able to
consistently perform in the altissimo range, up to G and
sometimes as high as C above high C. Students, therefore,
often tend to be discouraged when they attempt high-register
playing, because many of them experience difficulty even with
a note as low as F above middle C; many, if not most, regard C
above high C as unattainable. This has a tendency to dampen
interest; many abandon the trumpet altogether for this reason.
To summarize the current status of trumpet players in general,
we might say that they fall into one of roughly four
categories:
- A
handful of professional specialists who can, with extreme
physical effort and very shallow-cupped mouthpieces, execute
the altissimo range up to C above high C, but whose tone is
harsh in the lower register.
- Perhaps
ten percent who can play up to about F above high C, again
with extreme effort and shallow-cupped mouthpieces; these
players may also have a less-than-ideal tone.
- Possibly thirty percent who can only play up to about
high C, also with extreme effort.
- The
remaining roughly sixty percent, mostly students, who can
only reliably play up to about G below high C, and then with
considerable difficulty.
Clearly
then, essentially all trumpet players are limited, burdened,
and/or compromised in some way by mouthpieces that are
presently available to them. And, despite attempts by
instrument and mouthpiece makers to solve these problems, none
to date has been successful. The state-of-the-art of
mouthpiece design has progressed essentially no further
regarding these particular problems than the two
rules-of-thumb stated earlier. What is needed is a new
mouthpiece design that will reduce the difficulty of
high-register playing for all trumpet players, students as
well as professionals. This new design should also extend a
player's upper register by a significant number of semitones,
ideally five or more. And at the same time, it should impose
only minimal restrictions on tone quality. The mouthpiece
described in the following text has been designed to meet
these demands.
The Asymmetric Mouthpiece
To facilitate understanding of how the Asymmetric
mouthpiece satisfies the design criteria just mentioned, it is
necessary to review the mechanism of sound production using a
brass-wind instrument mouthpiece, to which end the following
remarks are given.
A popular misconception about brass instrument sound
production is that because sound is produced by a performer's
tensed, vibrating lips, pitch can be raised by increasing
tension in this lip tissue. We can see, however, using
elementary physical analysis, that increased tension alone in
the performer's lip tissue is insufficient to provide the lip
vibration frequency required to execute the complete range of
frequencies expected from a brass-wind instrument. The horn,
for example, produces about four usable octaves. Raising a
pitch by one octave doubles its frequency; four octaves raises
it sixteen fold. If we assume that all physical parameters
such as lip elasticity, mass etc. are constants, and tension
and frequency alone are allowed to vary, we can, using the
elementary equation for frequency vs. tension in a simple
vibrator, express the ratio of highest to lowest tension
as,
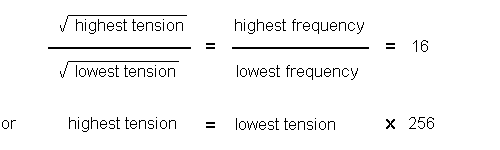
so that even if the lowest tension were only a few
ounces, the highest tension would be over thirty pounds and
would surely rupture soft lip tissue. Thus, we can safely
conclude that lip tissue tension alone cannot produce a four
octave range. What then, we might ask, is the supplementary
mechanism that enables four octaves to be played?
The facts are that although higher frequencies do
depend to some extent on increased lip tissue tension, the
major causal mechanism at work here is a reduction in the
effective vibrating mass of the upper lip. This reduction is
caused by the lower lip in the following way. When the
performer wishes to raise the pitch - whether realizing it or
not - he compresses the bottom lip upward against the top lip.
This upward compression has the effect of partially
immobilizing the upper lip and thus reducing its effective
vibrating mass. When the mass of a vibrator is reduced, the
frequency of vibration increases, and the pitch becomes
higher.
This effect is seen with other vibrators, such as a
violin string. To raise the pitch, a violinist shortens the
string by pressing it down against the neck with his finger.
The only portion that is then free to vibrate lies between his
finger and the bridge; this part contains less mass than the
complete string with no finger down to shorten it. Thus, the
lighter, shorter string has a higher pitch. The tension in the
string is essentially the same, with or without shortening. A
brass player's two lips function together much like the violin
string and the violinist's finger. To play the complete range
of the trumpet by relying on changing lip tension alone would
be like playing the violin with one open string, and
constantly changing the pitch using the tuning peg only -
clearly an impossibility! When we change lip tension, it's
like changing violin strings. This is a gross change (there
being four strings), and several pitches are available with
one tension, just as several pitches are available with one
string. When we push our bottom lip up when playing the
trumpet, it's like pushing our finger down when playing the
violin. Both a tension change and a mass change are effected,
but the mass change is clearly more important. It's necessary
to remember throughout this discussion, that if too much
pressure is applied to the lips via the mouthpiece, control is
lost and high range will be impossible, so we must concentrate
on "keeping a thick lip!" i.e., keeping sufficient lip tissue
between the mouthpiece and our teeth. Think of any required
"pressure" as vertical pressure between the lips,
rather than horizontal pressure (mouthpiece against the
lips).
Experimental studies (ref. Henderson) have verified
that the upper and lower lips of a trumpet player function in
distinct and different ways. In these studies, the upper lip
function was shown to be to vibrate back and forth (opening
and closing the embouchure) so as to admit consective puffs of
air into the mouthpiece, thus creating the alternating air
compressions and rarefactions required for sound production.
The principal function of the lower lip was shown to be to
press upward against the upper lip so as to control the
frequency of the upper lip's vibration by reducing, to varying
degrees, its effective vibrating mass. Having discussed this
concept of embouchure mechanics, I would now like to review
brass-wind instrument mouthpiece geometry as it relates to the
theory that was developed from systematic experimental
studies, along with developmental prototypes, to arrive at and
support the Asymmetric mouthpiece concept.
If we examine currently available brass-wind
mouthpieces, we find without exception, that they are radially
symmetric. This suggests that manufacturers may currently
believe that although the top and bottom lips have apparently
different physical structure, and although they perform
strikingly different functions, a mouthpiece can function well
without taking this into account; i.e., all commercially
available, radially symmetric mouthpieces do not acknowledge
either physical or functional differences between upper and
lower lips. We note, in contrast, that this is decidedly not
the case with reed instruments, such as the clarinet or
saxophone. With these instruments, the mouthpieces are highly
asymmetric and are designed specifically to accommodate both
physical and functional upper and lower lip differences.
Another possible explanation for brass mouthpiece symmetry is
that manufacturers may possibly not be aware of, or place any
importance on, the embouchure mechanics discussed above. But
the most likely explanation might be that mouthpieces have
always been made this way. Historically, the first "horns"
were, in all likelihood, animal horns with the small tip cut
off. Since then, the natural symmetry of the animal horn has
prevailed. Also, mouthpieces are turned on lathes, and this
mode of manufacture possibly tended to perpetuate the notion
of symmetry as being required or even ideal. At any rate,
radial symmetry has never been questioned, with specific
regard to the differing lip functions explained above, until
now.
Conjecturing that a mouthpiece cup could possibly
respond differently to top and bottom lips as well as to cup
depth, prototypes were made and experiments were performed
using an orthogonal composite fractional factorial regression
model (refs. Davies and Lynch) in which the curvature of the
top half of the cup, the curvature of the bottom half of the
cup, and the cup depth were treated as independent variables.
Optimization of the resulting performance response equation
showed the ideal mouthpiece to have a concave upper half and a
convex lower half. These experiements, along with several
subsequent prototypes made to explore and develop this
configuration, let to the following theoretical explanation
for the experimental results.
Let us assume that at some arbitrary frequency, a
player's bottom lip is exerting an upward force sufficient to
ensure that the effectively correct mass of upper lip tissue
will be vibrating to produce this frequency. As the player
attempts higher and higher frequencies, eventually he attains
the maximum amount of upward push that he is capable of
exerting and at that point is playing the highest pitch that
he is capable of producing. We now consider the bottom lip in
more detail.
The portion of the bottom lip tissue that lies inside
the boundary of the mouthpiece rim surface is rigidly
constrained on one side by the player's lower teeth. This
portion is also further rigidly constrained on its lateral and
bottom sides by the mouthpiece rim surface. It is not,
however, constrained on its front surface, which faces into
the mouthpiece cup, nor is it rigidly constrained on its top
surface, which is being pushed upward by the player against
his top lip. This upward push is caused by contracting the lip
muscles, especially those muscles that control the lower lip.
The lip tissue then is bulging upward and forward, the only
directions in which it is not rigidly confined. The upward
component of the bulge is producting the required upper lip
immobilization, and the forward component of the bulge is
causing lower lip tissue to enter the mouthpiece. This forward
bulge is contributing no constructive or significant action
except to reduce the cup volume slightly, which is producing a
slight to negligible effect on intonation and tone quality.
With this in mind, we now consider an alernative geomtery for
the bottom half of the cup.
If the lower half-cup surface edge nearest to the
bottom lip were made sufficiently convex, that portion of the
lower lip tissue intruding into the cup would now tend to be
pushed backward toward the player when it encountered this
convexity. The lower lip tissue then, being an elastic
container (lip tissue) filled with an essentially
incompressible fluid (blood), would act much like a balloon
filled with water and would accommodate this additional
compression by bulging even further in the only remaining
unconstrained direction (that direction for which there is no
rigid constraint), namely toward the upper lip. This
additional upward push would then result in additional upper
lip immobilization and therefore in an increase in upper lip
vibration frequency; i.e., highter pitch. Prototypes have
shown a typical increase in range due to this mechanism of as
much as seven semitones. Furthermore, because of the generally
convex contour of the leading edge of this lower convex
half-cup, the action of the mechanism is a progressive and
continuously increasing one with pitch; i.e., it has little to
no effect in the middle and low registers where lip intrusion
is negligibly small, and a gradually increasing effect with
frequency into the higher range where air pressure is higher
and the associated increased mouthpiece pressure against the
lips and increased muscular contraction normally causes larger
lower lip intrusions. Thus, the leading convex lower surface
not only extends a player's high-range capability but makes
all high-range playing easier.
Introducing the convexity into the lower half cup
surface, by itself, would reduce normal overall cup volume.
Without compensating for this reduction, tone would tend
toward the brassiness of shallow conventional-cup mouthpieces.
This cup volume reduction can be compensated for, however, by
enlarging the upper concave portion of the cup. For example,
if we know that a particular symmetric cup volume will produce
a particularly desirable tone quality, then instead of
reducing that volume by making the cup shallower in order to
obtain high-range capability (as is currently done, and
thereby destroying the tone), we instead spatially
redistribute this particular cup volume by making the bottom
surface convex and the top surface sufficiently concave. This
conforms with the experimentally derived ideal having concave
upper and convex lower half-cups. It has been shown that total
cup and backbore volume, rather than the particular shape of a
cup, tends to determine tone quality and playing properties
for a given player (ref. Benade). Thus, in this case, the
Asymmetric cup would have essentially the same total cup
volume as the symmetric cup, and the tone quality would remain
unimpaired. But, increased range and an overall ease of high
playing would be gained over the symmetric cup
mouthpiece.
It should be noted that any symmetric form of lower lip
restrictor would also restrict the upper lip and inhibit
vibration of this lip. And, even if such a restrictor were
relatively small in width, it would also reduce the span of
the cup for the upper lip. But the full span of the cup is
required for the upper lip lest the vibrating mass be
over-restricted and clean attack compromised. Both
calculations and prototypes have shown that even with
mouthpiece vertical respositioning, the tradeoff is such that
bottom lip performance is enhanced by the restrictor, but
simultaneous top lip restriction would impair its performance.
Thus, asymmetry is required. It should also be noted that
players using incorrect mouthpiece vertical positioning (other
than the generally accepted 1/3 on the top lip and 2/3 on the
bottom lip) will be unable to use the Asymmetric mouthpiece
successfully. Players who position the mouthpiece "half on
each lip" for example or more on the top than the bottom will
experience the convexity as an obstruction to the air stream.
Most players, however, use the "2/3 bottom, 1/3 top" position,
this having been experienced for the last hundred years (see,
for example, Arban's method) as more advantageous for higher
register playing. Although the reason for this is clearly that
much less top lip has to be immobilized, this was only known
by experience prior to the last 50 years. Other positions
decidely handicap the player unnecessarily.
Summary
The Asymmetric's cup design can add up to one-half
octave of high range capability and make all notes in the high
range generally easier to produce, and do this with no loss of
tone quality. The Asymmetric cup mouthpiece discussed herein
is therefore significantly and undeniably superior to radially
symmetric mouthpieces. Furthermore, the theory underlying this
concept is substantiated by prototypes and systematically
obtained experimental data and does not rely on cut-and-try
efforts.
The Asymmetric mouthpiece is used in exactly the same
manner as a symmetric mouthpiece with one small but important
exception; the Asymmetric must be inserted into the trumpet
with the convex portion of the cup surface down, so as
to be substantially nearer the bottom lip than the top lip of
the performer. Once installed with this orientation, no other
special consideration is required because the mouthpiece does
not rotate in the instrument when playing. Tests showed that
as much as ten degrees of rotation, clockwise or
counterclockwise could be tolerated without appreciably
impairing the Asymmetric mouthpiece's efficacy. Also, the
orientation of the trumpet's slides, valves, and other
structure vis-a-vis the mouthpiece's axial orientation
provides the player with an instant visual confirmation of the
mouthpiece's axial orientation when playing. This orientation
will remain essentially constant, because the performer's hand
positions when playing must remain essentially constant to
ensure unimpaired valve manipulation.
References
Henderson, H.W. An Experimental Study of Trumpet
Embouchure. J.A.S.A., Vol. 13, pp. 58-64, July
1942.
Davies, O.L. The Design and Analysis of Industrial
Experiments. Hafner Publishing Co., 1956.
Lynch, J.H. A Systematic Approach to Model
Development by Comparison of Experimental and Analytical
Regression Coefficients. NASA TM-X 1797, May
1969.
Benade, A.H. Fundamentals of Musical Acoustics.
Oxford University Press, pp. 414-418, 1976
|